Explore solar panel parts and components. Learn how to go off-grid with solar power and create your own photovoltaic system.
If you’re serious about off-grid power, you won’t be surprised to hear that designing for off-grid photovoltaic (PV) systems is a little more complicated than it is for grid-tied systems — but it’s probably still simpler than you think. While the two types of systems have a lot in common, an off-grid system incorporates three extra elements — batteries, a charge controller, and a generator. Although, to counteract this, there are two things that you won’t have to worry about: meters and hassles with the utility company (not to mention utility bills).
Off-Grid Solar Basics
To appreciate the primary difference between off-grid and grid-tied PV systems, imagine you’re planning to take everyone in your household on a long camping trip. One option is to go car camping, where you park your vehicle right at the campsite. You’ll spend your days and nights outdoors, but if you run out of food, beer, toilet paper, or other essentials, you’ll have plenty of backup supplies packed in the car, just a few steps away.
Your other option is a wilderness trip, where you carry everything you need to survive deep into the woods in a backpack. If you plan carefully, and make sure nobody eats or drinks too much, you’ll do just fine. But if supplies run low, you’ll have to cut back on your consumption. And if your supplies run out, they run out. You’re camped too far from the car to hike back, and you didn’t fill it with extra supplies anyway.
You get the idea. Planning an off-grid PV system, like wilderness camping, requires more care and thoughtful examination of what you need on a daily basis. The only thing missing from the comparison, in the off-grid scenario, is a generator for replenishing the energy supply if things get dire. In this instance, you can think of the generator as a fishing pole in our camping metaphor.
It’s a simple matter of supply and demand. Sizing an off-grid system should start with a careful examination of your household electrical demand — your daily electrical loads. (You’ll learn how to calculate loads in “Calculating Loads and Days of Autonomy.”)
Now, let’s take a look at the basic elements of an off-grid PV system.
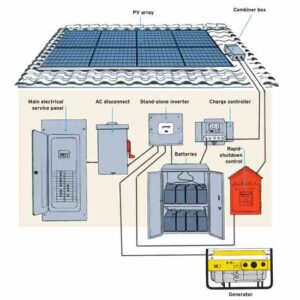
Off-Grid Solar Components
PV array. The modules and module support structure are the same for off-grid and grid-tied systems, but with an off-grid design, the module series-strings tend to be much smaller, typically with strings of three or four modules each. (We’ll discuss this more in “Array Layout.”)
Rapid shutdown. Off-grid systems use string inverters (not microinverters), which means they need a dedicated system to meet rapid-shutdown requirements. This usually takes the form of a rapid-shutdown control switch that communicates with a disconnecting combiner box at the array, as with a standard grid-tied string-inverter system.
Charge controller and batteries. In an off-grid system, the solar-generated DC power from the array passes through a charge controller on its way to the batteries. The charge controller regulates the flow of power and steps down the voltage to the proper level for the battery bank design (you can learn more about batteries for solar installations at The Best Batteries for Your Off-Grid Battery Bank). The charge controller is essential for safe charging and for battery health. It turns on when the battery voltage is low and the battery bank needs charging. It turns off when the battery voltage is high and the batteries are fully charged.
DC to AC inverter. The stored DC power in the batteries runs through an inverter to be converted to AC power for use in the household electrical system. Remember that inverters for off-grid PV systems are called “stand-alone inverters,” while those for grid-tied systems are called “grid-tie inverters.” Conventionally, microinverters are not used for off-grid systems because the batteries require DC power, and microinverters convert to AC at the module. However, some AC-coupled systems use microinverters (in a setup that’s sometimes called a “microgrid”), but this entails unnecessary complications for most single-home systems.
AC disconnect. On the AC side of things, off-grid systems have only an AC disconnect and the home’s main electrical service panel, both of which are the same as those used with grid-tied systems. Of course, no production meter or utility meter will be required, since you won’t be connected to the utility grid.
Generator. Off-grid households that rely on solar power almost always have a generator for backup power when battery storage levels are low, the sun isn’t shining, or both.
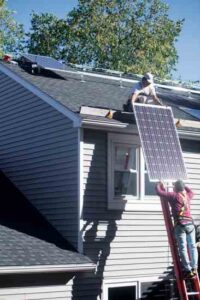
Calculating Loads and Days of Autonomy
Your electrical loads are the cumulative totals of all daily electrical usage in your house. Calculate your loads by multiplying the wattage of each electric appliance and device by the average amount of time it’s used in a day. For appliances that are used less regularly, such as a clothes washer, calculate the daily average based on your weekly use. For example, if you run the washer 3.5 hours per week, use a daily figure of 0.5 hour (3.5 ÷ 7 = 0.5). Add up the usage of all appliances to find the daily total kilowatt-hours (kWh) used in your home. This number will be your minimum daily goal for AC power produced by your PV system. During the design process, you can use the National Renewable Energy Laboratory’s online PVWatts Calculator to determine a DC system size for reaching this goal.
The chart to the left lists some common household appliances and devices and their approximate wattages and daily usage times (annual averages). It offers just a snapshot of a household with fairly efficient appliances. As you probably know, there are manufacturers that specialize in ultra-efficient appliances for off-grid homes. Investing in just one ultra-efficient major appliance, such as a refrigerator or a stove, can make a huge difference in your household’s daily load.
Determine the wattage of your own appliances by looking on the manufacturer’s nameplate. If it doesn’t list the wattage but gives you the amps, multiply that number by 120 for standard-voltage appliances, or by 240 for dryers, electric stoves, and other high-voltage appliances. For example, if an electric fan is rated for 3 amps, its wattage is 3 amps × 120 volts = 360 watts.
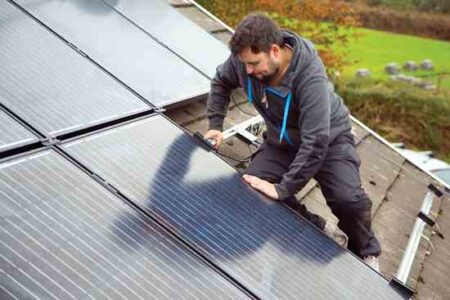
Wattage is the measure of instantaneous power consumption of an appliance — in the same way that it’s the measure of power output from a PV module at a given point in time. To convert wattage to actual energy usage, you have to add a factor of time: a 360-watt fan running for 3 hours uses 1,080 watt-hours. Divide the watt-hours by 1,000 to find the kilowatt-hour (kWh) usage: 1,080 ÷ 1,000 = 1.08 kWh. Use the kWh values for totaling your household electrical loads.
“Days of autonomy” is a critical design factor for off-grid systems. It answers the question “How many days can I go with no sun?” In other words, if it’s the dead of winter, and the forecast shows nothing but snow or dense cloud cover for the near future, how long do you want your batteries to meet your daily loads before you run out of power?
Days of autonomy are determined by the capacity of your battery bank. You can choose the number of days you like, but the standard target is three days, assuming the system includes an electrical generator for backup power during extended periods of cloudy weather. A three-day target seems to strike the right balance between cost and benefit for most off-grid homeowners. There’s no rule against designing for more days; it just requires more money for a larger battery bank. You’ll apply your target number of days of autonomy when sizing your battery bank.
Solar Array Layout
The design for the array (including the modules and module support structure) is the same for grid-tied and off-grid systems. To clarify: The physical layout of the modules and all the support structure calculations can be exactly the same, but the electrical layout is different.
With a grid-tied string-inverter system, the length of the series-strings is ultimately limited by the maximum allowed input DC open-circuit voltage (Voc) rating of the string inverter, and 8 to 12 modules in a string is common. With off-grid systems, the series-strings are limited by the charge controller. Most charge controllers can handle strings of no more than three or four modules in series. For example, if you have 12 modules total, you would typically wire the array in four parallel strings of three modules each. Many newer charge controllers can handle four modules in series (three parallel strings of four modules each for a 12-module array), and some can do several more in series, with ratings up to 600 volts max open-circuit voltage.
Choose the charge controller rating that best fits your array size. Remember that the physical layout of the modules doesn’t have to match the electrical layout. For example, your 12-module array could be laid out in two physical rows of six modules each and still be wired as four strings of three modules each.
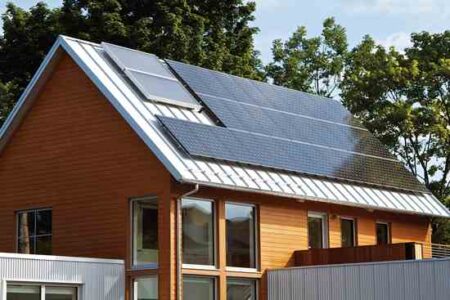
Charge Controllers
Charge controllers are needed for off-grid PV systems because the module strings put out much higher voltage than the batteries can handle. The charge controller receives the higher voltage (under ideal conditions) from the array and delivers the correct lower voltage to the batteries. In addition, it regulates the amount and rate of charging that the batteries require at all times.
Charge controllers come in many types, with different ranges of cost and capability. The only type that’s highly recommended for an off-grid PV system is one with maximum power point tracking (MPPT), which can accept an input voltage from the array that’s higher than its output voltage (that is, the voltage that goes to the batteries), as discussed previously. The other type is a fixed-voltage charge controller, which requires that the array voltage be the same as the battery voltage. This type is used only with very small PV systems and usually isn’t suitable for home power systems.
MPPT capability on a charge controller is similar to MPPT with inverters (and DC optimizers). In this case, the charge controller (not the inverter) constantly monitors the voltage and current of the modules and adjusts the levels as needed to maintain the maximum power point. Because the most dramatic adjustments are needed during less-than-ideal sun conditions, extreme temperature conditions, and low-battery conditions, MPPT charge controllers really earn their keep in cloudy weather, when the modules are partially shaded, or during any extremes in solar irradiance or temperature.
Generators
Most off-grid PV systems include a generator for backup power when the PV system can’t meet the demands of the household electrical loads, usually during the low-light days of winter or extended cloudy periods. Generators are also useful for equalizing batteries and for powering (or helping to power) significant loads that would otherwise use a lot of stored power in the batteries.
You’ll have many factors to consider when choosing a generator, including the size of the battery bank, the household loads, and the AC output of the PV inverter. If your house has only 120-volt loads, you can use a generator that outputs at only 120 volts. But if your house has some 240-volt loads, the generator must have both 120- and 240-volt output. In this case, it’s important to check the generator’s output ratings carefully to understand the actual output at both 120 and 240 volts. As with stand-alone 120/240-volt inverters, there may be a reduction in output when drawing 120-volt power. Generators typically are sized to cover a little more power than the PV inverter’s full charging capacity, plus any household loads that may run concurrently with battery charging.